Home Planet: Our Blue Oasis
ยฉ EUMETSAT/ESA
Earth, our planetary abode, takes the third position in our solar system, ranking as the fifth most voluminous planet. It is surpassed in size by the four colossal gas giants โ Jupiter, Saturn, Uranus, and Neptune โ but surpasses the other three terrestrial planets, namely Mercury, Mars, and Venus.
While scientists persist in their search for signs of life beyond Earth, our homely planet remains the sole celestial body where we have confirmed the existence of living organisms. This is made possible by Earth’s aqueous nature, rendering it the solitary entity in the Solar System to harbor liquid surface water.
Almost all of Earth’s water is contained in its global ocean, spanning 70.8% of Earth’s surface. The remaining 29.2% is land, which consists mainly of continental landmasses mostly on Earth’s land hemisphere. While Earth’s land is also covered by considerable amounts of water, predominantly in the form of large sheets of ice covering polar land, containing more water than Earth’s groundwater, lakes, rivers and atmospheric water together, most of Earth’s land is covered by vegetation.
When observed from another celestial body in our solar system, Earth would present itself as a luminous, bluish orb. Through a powerful telescope, one would discern its atmospheric characteristics, particularly the churning, white cloud formations of midlatitude and tropical storms, arranged in distinct latitudinal bands around the planet. The polar regions, too, would shine in a brilliant white hue, due to the cloud cover above and the expanse of snow and ice below. Below the ever-shifting cloudscapes, one would glimpse the much deeper blue-black expanses of the oceans, occasionally interrupted by patches of tawny desert terrain. The verdant landscapes that host most human habitation would prove challenging to discern from space.
Not only do they account for a relatively small portion of the total landmass, which itself constitutes less than one-third of Earthโs surface, but they are frequently veiled by clouds. Throughout the seasons, some changes in the storm patterns and cloud belts on Earth would be observed. Also prominent would be the growth and recession of the winter snow cap across land areas of the Northern Hemisphere.
Earth’s surface is traditionally categorized into seven distinct continental masses: Africa, Antarctica, Asia, Australia, Europe, North America, and South America. These continents are encompassed by five major bodies of water: the Arctic, Atlantic, Indian, Pacific, and Southern Oceans.
Nonetheless, it is practical to analyze different segments of Earth in terms of concentric, roughly spherical layers. Progressing from the innermost to the outermost regions, these encompass the core, the mantle, the crust (inclusive of the rocky surface), the hydrosphere (primarily comprising the oceans, which occupy low-lying areas in the crust), the atmosphere (itself partitioned into spherical strata like the troposphere, where weather phenomena occur, and the stratosphere, housing the ozone layer safeguarding Earth’s surface and its inhabitants from the Sun’s ultraviolet rays), and the magnetosphere (an extensive region in space where Earth’s magnetic field exerts dominance over electrically charged particles originating from the Sun).
ABOUT THE PLANET – EARTH
The planet has a diameter of roughly 8,000 miles (13,000 kilometers) and is primarily round because gravity generally pulls matter into a ball. But the spin of our home planet causes it to be squashed at its poles and swollen at the equator, making the actual shape of the Earth an “oblate spheroid.”
INTERNAL STRUCTURE
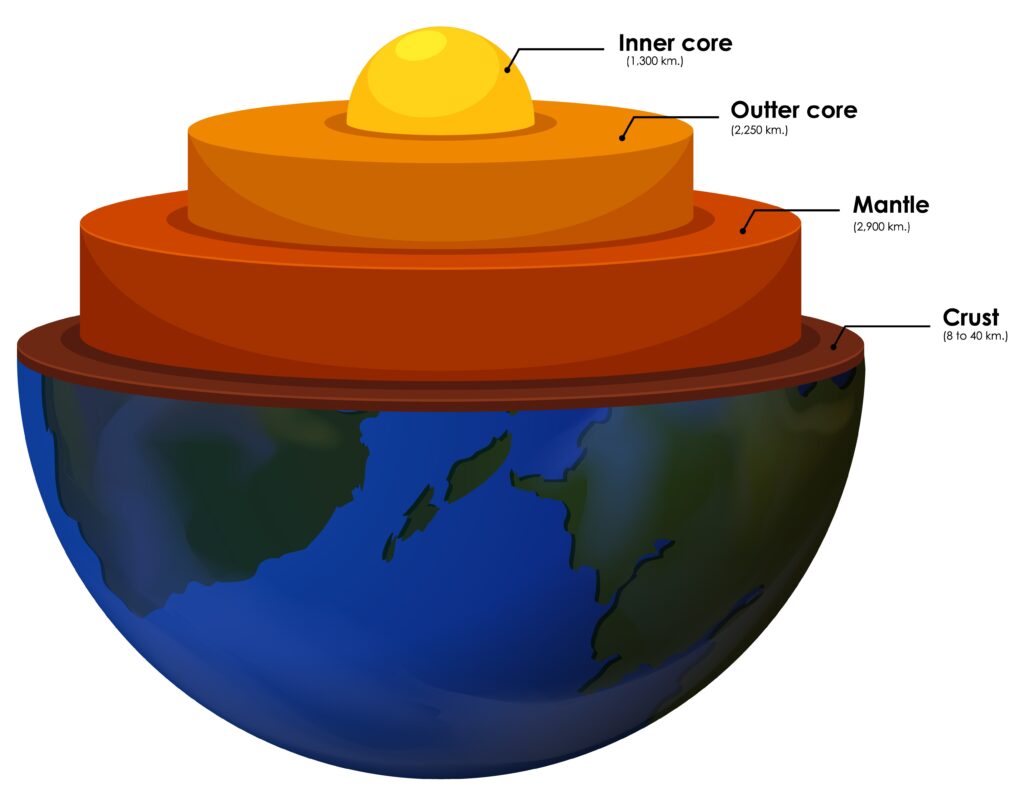
Similar to the other terrestrial planets, Earth’s interior is stratified based on chemical and physical (rheological) attributes. Over 90 percent of Earth’s mass is constituted by iron, oxygen, silicon, and magnesiumโelements capable of creating crystalline minerals called silicates. However, in both chemical composition and mineralogical structure, Earth exhibits significant heterogeneity.
Earth can be divided into the crust, mantle, outer core, and inner core. The geologic component layers are at increasing depths below the surface:
CRUST
The Earth’s crust is the outermost layer and is divided into two types: the continental crust and the oceanic crust. The continental crust is thicker and less dense, composed mainly of granite rocks. The oceanic crust is thinner and denser, primarily composed of basaltic rocks. The crust is the layer where we live and where most geological processes, such as erosion and volcanic activity, take place.
MANTLE
Below the crust lies the mantle, which is much thicker and extends to a depth of about 2,900 kilometers (1,800 miles). The mantle is composed of solid rock that can flow over long periods; a property known as “mantle convection.” This convective movement of material is responsible for driving the motion of tectonic plates on the Earth’s surface. The mantle is divided into the upper mantle and the lower mantle, with the lower mantle being more rigid due to the high pressures at that depth.
OUTER CORE
Beneath the mantle is the outer core, which is a liquid layer composed mainly of iron and nickel. This layer is responsible for generating Earth’s magnetic field through the process of convection-driven motion of the molten metals. The outer core extends from a depth of about 2,900 kilometers (1,800 miles) to approximately 5,150 kilometers (3,200 miles).
INNER CORE
The innermost layer of Earth is the inner core, composed primarily of iron and nickel in a solid state. Despite the immense pressure, the inner core remains solid due to the high temperature. The inner core extends from a depth of about 5,150 kilometers (3,200 miles) to the center of the planet, which is estimated to be around 6,371 kilometers (3,959 miles) from the surface.
The transitions between these layers are marked by changes in physical properties, such as density, composition, and state (solid or liquid). The movement of material within the Earth’s layers drives various geological processes, including plate tectonics, volcanic activity, earthquakes, and the generation of the magnetic field.
SURFACE

Roughly 70.8% of the Earth’s surface is enveloped by water, leaving about 29.2% comprising the seven continental landmasses. Beneath the expanse of oceans and the layers of soil and vegetation on the continents lies the bedrock that forms the Earth’s outer surface. This rugged, rocky crust took shape as molten lava cooled roughly 4.5 billion years ago. This crust is divided into numerous substantial plates, known as tectonic plates, which shift at a gradual pace relative to one another. The world’s mountain ranges emerged from the collision of these plates, forcing their edges upward. Many other surface characteristics arise from the movements of these tectonic plates.
The Earth’s surface features stem from both constructive and destructive forces. Constructive forces facilitate the growth of landforms, as seen in the birth of a new volcano, creating fresh terrain. Destructive forces, on the other hand, erode and wear down landforms. The gradual processes of mechanical and chemical weathering, as well as erosion, labor tirelessly to transform once towering mountains into gently sloping plateaus. Roughly 250 million years in the past, most of the landmasses were interconnected.
The rocky stratum below the Earth’s surface, known as the crust, encompasses both continents and ocean basins. Its thickness varies, ranging from 35 to 70 kilometers on continents to 5 to 10 kilometers in ocean basins.
The tectonic plates float atop the semi-molten asthenosphere, which constitutes the lower mantle of the Earth. Earthquakes, volcanoes, the formation of mountains, and the creation of oceanic trenches all occur along plate boundaries. These plates are in a perpetual state of motion. The capacity for tectonic plates to shift is attributed to the higher strength and lower density of the Earth’s lithosphere compared to the underlying asthenosphere. Their motion is steered by the dissipation of heat from the Earth’s mantle.
Lateral variations in density within the mantle trigger convection, which is translated into plate movement through a combination of frictional resistance, downward pull at subduction zones, and disparities in the topography and density of the crust, leading to variations in gravitational forces.
CHARACTERISTICS
MASS | 5.972168ร1024 kg |
VOLUME | 1.08321ร1012 km3 |
SURFACE AREA | 510,072,000 km2 |
MEAN RADIUS | 6371.0 km |
SURFACE PRESSURE | 101.325 kPa |
DENSITY | 5.5134 g/cm3 |
ESCAPE VELOCITY | 11.186 km/s |
SURFACE GRAVITY | 9.80665 m/s2 |
ABSOLUTE MAGNITUDE | โ3.99 |
NATURAL SATELLITES | 1 |
RINGS | NO |
MEAN TEMPERATURE | 14.76 ยฐC |
SEMI-MAJOR AXIS | 149,598,023 km |
ORBIT PERIOD | 365.256363004 days |
PERIHELION | 147,098,450 km |
APHELION | 152,097,597 km |
MEAN ORBITAL VELOCITY | 29.78 km/s |
MAXIMUM ORBITAL VELOCITY | 30.29 km/s |
MINIMUM ORBITAL VELOCITY | 29.29 km/s |
ORBIT INCLINATION | 23.5ยฐ |
ORBIT ECCENTRICITY | 0.0167 |
SIDEREAL ROTATION PERIOD | 23.92 hours |
LENGTH OF DAY | 24 hours |
ORBIT AND ROTATION

As Earth revolves around the Sun, it also rotates on an imaginary axis extending from the North Pole to the South Pole, creating a spinning motion. It takes the planet 23.934 hours to complete a rotation on its axis and 365.26 days or one sidereal year to complete an orbit around the Sun โ our days and years on Earth are defined by these gyrations.
Earth’s average orbital distance is about 150 million km (93 million mi), which is the basis for the Astronomical Unit and is equal to roughly 8.3 light minutes or 380 times Earth’s distance to the Moon. The orbital speed of Earth averages about 29.78 km/s (107,200 km/h; 66,600 mph), which is fast enough to travel a distance equal to Earth’s diameter, about 12,742 km (7,918 mi), in seven minutes, and the distance to the Moon, 384,000 km (239,000 mi), in about 3.5 hours.
Earth happens to orbit the Sun within the so-called “Goldilocks zone,” where temperatures are just right to maintain liquid water on our planet’s surface. It’s orbit is not a perfect circle, but rather a slightly oval-shaped ellipse, similar to the orbits of all the other planets in our solar system. In early January, our planet is slightly nearer to the Sun, while in July, it’s a bit farther away. However, this difference in proximity has a far smaller impact on surface temperatures than the tilt of Earth’s axis.
Earth’s axis of rotation is tilted about the ecliptic plane, an imaginary surface through the planet’s orbit around the Sun. This means the Northern and Southern Hemispheres will sometimes point toward or away from the Sun depending on the time of year, and this changes the amount of light the hemispheres receive, resulting in the changing seasons.
Earth’s rotation period with respect to the Sun, known as a solar day, lasts for 86,400 seconds of mean solar time (equivalent to 86,400.0025 SI seconds). Due to tidal deceleration, the solar day has gradually lengthened since the 19th century, resulting in each day varying between 0 and 2 milliseconds longer than the average solar day.
In 2020, scientists observed a noteworthy acceleration in Earth’s rotation speed, after years of it consistently slowing down from the 86,400-second mark. On June 29, 2022, Earth completed a full rotation in just 1.59 milliseconds less than 24 hours, establishing a new record. This trend has prompted global discussions among engineers about the potential implementation of a ‘negative leap second’ and other timekeeping adjustments.
This increase in speed is believed to stem from a range of factors, including the intricate movements of Earth’s molten core, oceans, and atmosphere, as well as the influence of celestial bodies like the Moon. Additionally, climate change may be contributing, as it leads to the melting of polar ice, which in turn affects Earth’s shape. The presence of substantial ice masses contributes to Earth’s oblate spheroid shape, with a bulge around the equator.
As these masses diminish, the poles rebound from the loss of weight, causing Earth to approach a more spherical shape. This, in turn, concentrates mass closer to its center of gravity, leading to an increase in rotational speed according to the principle of conservation of angular momentum.
ATMOSPHERE
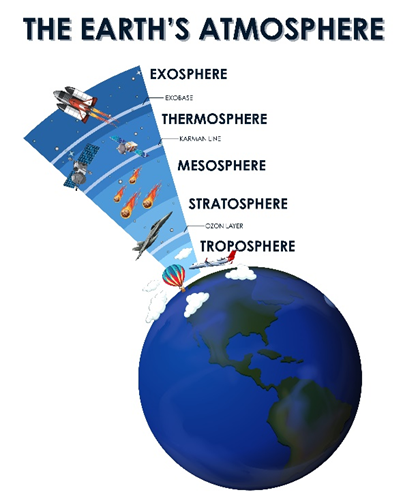
Earth is encompassed by a relatively slender atmosphere, often referred to as air, comprised predominantly of molecular nitrogen (about 78 percent) and molecular oxygen (approximately 21 percent). Additionally, trace amounts of gases like argon (nearly 1 percent), variable water vapor (averaging around 1 percent but subject to significant fluctuations in time and location), carbon dioxide (0.0395 percent or 395 parts per million, currently on the rise), methane (0.00018 percent or 1.8 parts per million, presently increasing), as well as other gases, coexist with minuscule solid and liquid particles suspended in the mix.
Among all the planets in our solar system, Earth stands alone in possessing an atmosphere rich in free oxygen, a crucial element for one of the planet’s other extraordinary features: the presence of life.
In its early stages, Earth’s atmosphere was composed primarily of hydrogen from the solar nebula. Over time, various factors like volcanism, life, and weathering instigated significant changes in the atmospheric composition. In recent times, human activities have also played a role in altering the atmosphere, contributing to phenomena like global warming, ozone depletion, and acid deposition.
The atmosphere boasts a total mass of approximately 5.15ร10^18 kilograms, with about three-quarters of this mass contained within an 11-kilometer (6.8-mile) span above the surface. As one ascends, the atmosphere gradually thins, and there’s no distinct demarcation between the atmosphere and outer space. Around 100 miles (160 kilometers) above Earth, the air thins to the point where satellites can move through with minimal resistance. Nevertheless, traces of the atmosphere can still be detected as high as 370 miles (600 kilometers) above the planet’s surface.
The atmosphere is divided into several layers based on temperature changes with increasing altitude:
TROPOSPHERE
This is the layer closest to the Earth’s surface and extends to an average altitude of about 8-15 kilometers (5-9 miles). It contains most of the planet’s weather phenomena and is where temperature generally decreases with altitude. The troposphere is delimited at its uppermost extent by the tropopause. This boundary is typically characterized by a temperature inversion, wherein a layer of warmer air lies above a colder one. In certain areas, it may also be demarcated by a region exhibiting consistent temperature with increasing altitude.
STRATOSPHERE
Beyond the troposphere lies the stratosphere, spanning approximately 15 to 50 kilometers (9 to 31 miles) in altitude. Within the stratosphere resides the ozone layer, a region that absorbs and disperses a substantial portion of the Sun’s ultraviolet (UV) radiation. At the upper reaches of the stratosphere, atmospheric pressure is roughly only 1/1000th of that at sea level. The stratosphere is characterized by a temperature increase as altitude increases. This warming effect is a result of the ozone layer absorbing ultraviolet radiation (UV) from the Sun, which in turn limits turbulence and mixing.
MESOSPHERE
The mesosphere is situated between about 50 and 85 kilometers (31 to 53 miles) above the Earth’s surface. It is the coldest layer of the atmosphere and is also where meteors burn up upon entering Earth’s atmosphere. As altitude increases, temperatures decline until reaching the mesopause, which signifies the upper boundary of this intermediate atmospheric layer. It is the coldest place on Earth and has an average temperature of around โ85 ยฐC (โ120 ยฐF; 190 K). Just below the mesopause, the air is so cold that even the very scarce water vapour at this altitude can condense into polar-mesospheric noctilucent clouds of ice particles.
THERMOSPHERE
This layer starts around 85 kilometers (53 miles) above the surface and extends to the exosphere. Despite its name, the thermosphere can have very high temperatures due to the absorption of high-energy solar radiation. The International Space Station circles within this realm. The thermopause, also known as the exobase, marks the lower extremity of the exosphere. Within the lower segment of the thermosphere, spanning from 80 to 550 kilometers (50 to 342 miles) above Earth’s surface, lies the ionosphere. While the temperature of the thermosphere progressively escalates with altitude and can reach up to 1500 ยฐC (2700 ยฐF), the molecules are so dispersed that its temperature in the conventional sense lacks substantial meaning.
EXOSPHERE
The exosphere is the outermost layer of the atmosphere and extends from the thermopause (also known as the “exobase”) at the top of the thermosphere to a poorly defined boundary with the solar wind and interplanetary medium. This layer primarily consists of exceedingly sparse amounts of hydrogen, helium, and a few denser molecules like nitrogen, oxygen, and carbon dioxide, particularly nearer to the exobase. The atoms and molecules are dispersed to the extent that they can traverse hundreds of kilometers without encountering one another.
The exosphere is situated at such a distance from Earth that meteorological phenomena are not viable. Nevertheless, Earth’s aurorasโthe aurora borealis (northern lights) and aurora australis (southern lights)โoccasionally manifest in the lower region of the exosphere, where they intersect with the thermosphere. Many artificial satellites and other objects in space orbit within the exosphere.
MAGNETIC FIELD

Earth’s magnetic field is a phenomenon generated by the movement of molten iron and nickel in the outer core of the planet. This magnetic field extends into space and forms a protective shield around the Earth, influencing a wide range of natural processes and even technological systems.
GENERATION
The Earth’s magnetic field is produced by a mechanism called the geodynamo. According to this theory, the movement of molten iron and nickel in the Earth’s outer core induces electric currents due to the convective motion of the liquid metal. These electric currents, in turn, generate a magnetic field. The rotation of the Earth also contributes to the dynamo effect, as the moving, electrically conducting fluids create a self-sustaining loop of magnetic field generation.
STRUCTURE
The Earth’s magnetic field is roughly similar to that of a bar magnet, with magnetic north and south poles. However, these magnetic poles are not aligned with the geographic poles (the points around which the Earth rotates). The magnetic north pole is located in the northern hemisphere but is physically opposite to the geographic north pole. Similarly, the magnetic south pole is opposite to the geographic south pole.
Magnetic declination is the angle between the geographic north (the direction toward the North Pole) and the magnetic north (the direction a compass needle points). This angle varies depending on your location on Earth’s surface. Magnetic inclination (or dip) refers to the angle that the magnetic field lines make with the Earth’s surface. The inclination is steeper near the magnetic poles and nearly horizontal near the equator.
Earth’s magnetic field has several important effects and functions as it has been used for navigation for centuries, as compasses align themselves with the magnetic field lines and the magnetic field acts as a shield, deflecting charged particles (solar wind) coming from the Sun. These particles would otherwise strip away the Earth’s atmosphere and pose a danger to living organisms.
However, it’s important to note that Earth’s magnetic field is not a constant or permanent feature. It has undergone numerous reversals in the past, where the magnetic north and south poles switch places. These reversals are recorded in the Earth’s rocks and have occurred irregularly throughout geological history.
GRAVITY
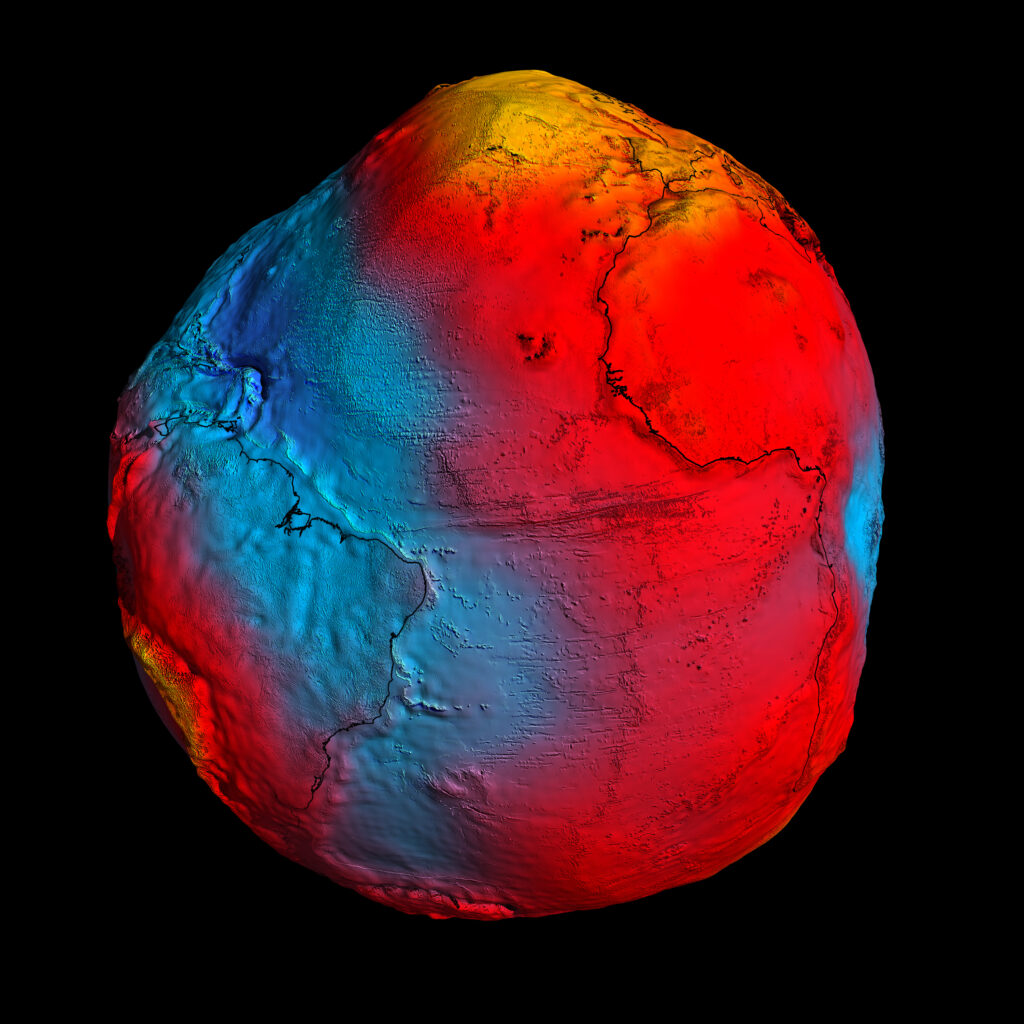
Earth’s gravitation is the unseen force that shapes our physical reality, defining the interaction between all matter on the planet’s surface and beyond. This universal force, stemming from the planet’s mass, draws everything towards its centre with an acceleration of approximately 9.8 meters per second squared (m/sยฒ). This force is responsible for the weight we experience, as objects on Earth’s surface are subject to the pull of gravity. From a pebble resting on the ground to a soaring skyscraper, all objects are bound by this force that keeps them anchored to the Earth.
Moreover, Earth’s gravitation extends its influence beyond its immediate surroundings. It governs the motion of celestial bodies, orchestrating the dance of the Moon, the orbits of satellites, and the gravitational interactions within the solar system and beyond. The phenomenon of tides, where oceans experience periodic rises and falls, is a direct consequence of the interplay between Earth’s gravitational force and the Moon’s pull.
Learn about Earth and Moon System here.
The understanding of Earth’s gravitation has transcended scientific exploration, finding its place in cultural, philosophical, and technological realms. It has guided navigation since ancient times, allowing sailors and explorers to traverse vast oceans and uncharted territories. The laws of gravitation elucidated by luminaries like Isaac Newton and Albert Einstein have revolutionized our comprehension of space, time, and the intricate workings of the universe.
In conclusion, Earth’s gravitation is a fundamental force that shapes our world in profound ways. Its reach extends from the smallest particles to the largest galaxies, weaving an intricate tapestry of interconnectedness throughout the cosmos. This force, often taken for granted, is at the heart of our daily experiences, scientific endeavours, and the fundamental truths that underlie the nature of our existence.
Previous Planet – “Venus“
Next Planet – “Mars“