Unraveling the Layers of the Sun
ยฉ NASA Goddard
Table of Contents
In the vast expanse of the cosmos, Sun’s Structure plays a pivotal role in our solar system, we must delve deep into its heart and explore the intricate layers. The Sun, as seemingly simple as it appears in our daytime sky, is a tapestry of complexity. From the searing inferno of its core to the shimmering beauty of its surface.
In this exploration, we embark on a journey to unravel the Sun’s structure, unveiling its inner workings and the layers that define its being. We will venture from the heart of the star, where nuclear fusion forges elements, to the brilliant surface, where sunspots and solar flares dance upon the stage of the photosphere. Along the way, we will encounter the mysterious chromosphere and corona, where the Sun’s outer atmosphere comes to life.
CORE
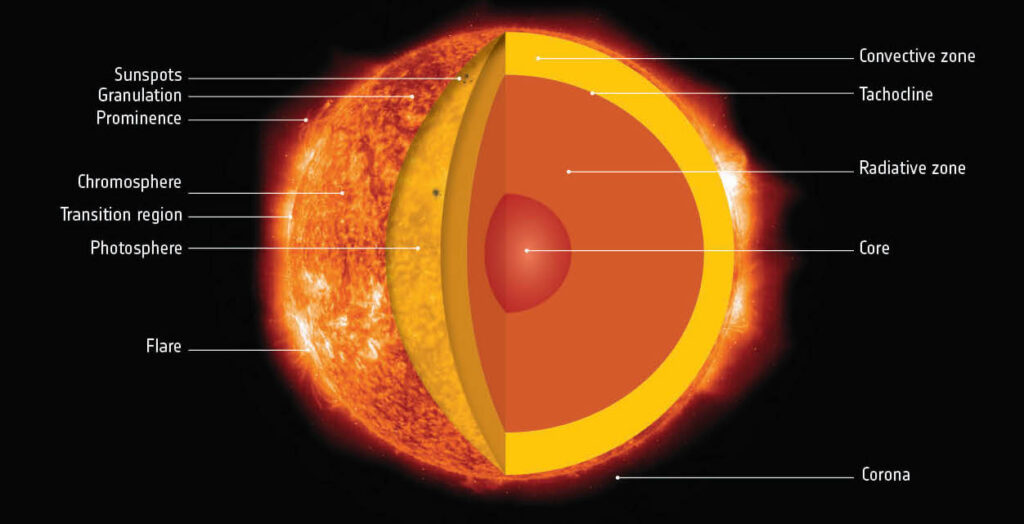
At the heart of the Sun’s structure lies its core, the innermost and pivotal region where the Sun’s energy production is driven. This core functions as the “engine” that fuels the Sun, hosting nuclear fusion reactions that have persisted for billions of years. This ceaseless process involves the transformation of hydrogen into helium, serving as the lifeblood of the Sun’s radiant energy, which, in turn, bestows upon our solar system the indispensable light and warmth on which it depends.
COMPOSITION
The core is primarily composed of hydrogen gas (about 74% by mass) and helium gas (about 24% by mass). These are the two lightest and most abundant elements in the universe.
NUCLEAR FUSION
The core is where the Sun’s energy is generated through a process called nuclear fusion. Specifically, the Sun undergoes a series of nuclear reactions known as the proton-proton chain, which converts hydrogen nuclei (protons) into helium nuclei.
TEMPERATURE
The core is incredibly hot, with temperatures reaching approximately 15 million degrees Celsius (27 million degrees Fahrenheit). These extreme temperatures are necessary to initiate and sustain the nuclear fusion reactions.
PRESSURE
The intense heat and gravitational pressure at the core create conditions where hydrogen nuclei collide with enough energy to overcome their mutual electrical repulsion, allowing them to fuse into helium nuclei. This process releases an enormous amount of energy in the form of photons (particles of light).
SIZE AND DENSITY
Within the framework of the Sun’s structure, the core encompasses roughly a quarter of the Sun’s overall radius, rendering it a relatively compact sector. Yet, despite its modest size in comparison to the broader Sun’s structure, the core exhibits an extraordinarily high density, attributable to the immense pressure and temperature it harbors. Remarkably, this density reaches approximately 150 times that of water, notwithstanding the fact that the core is predominantly composed of gaseous hydrogen and helium.
RADIATIVE ZONE
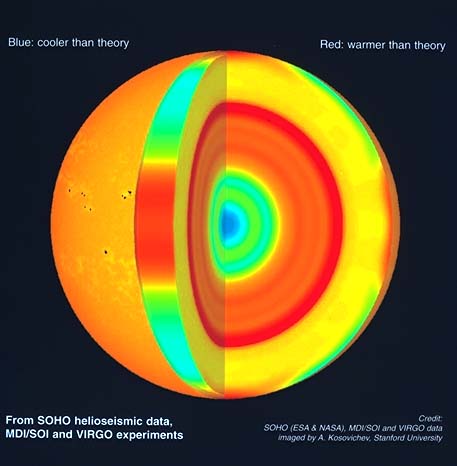
Within the Sun’s structure, the radiative zone emerges as a pivotal region, situated between the core and the convective zone. Its vital function is to facilitate the transfer of energy from the Sun’s core to its outer layers. The primary means of energy conveyance in the radiative zone occurs through the emission and absorption of photons, a process known as radiation.
POSITION
In the hierarchy of the Sun’s structure, the radiative zone lies immediately above the central core, stretching outward to encompass approximately 70% of the Sun’s total radius. This zone commands a substantial segment within the Sun’s structure.
PHOTON INTERACTIONS
In the core, nuclear fusion reactions produce extremely high-energy photons, primarily in the form of gamma rays. These photons travel outward but are frequently absorbed and then re-emitted by atoms within the dense plasma of the radiative zone.
RANDOM WALK
Photons experience a “random walk” as they move through the radiative zone. They are absorbed and re-emitted countless times as they interact with atomic nuclei and electrons in the plasma. This process is analogous to a game of “pinball,” with photons bouncing around in random directions. Due to the random walk and multiple interactions, photons take a long timeโthousands to millions of yearsโto traverse the radiative zone and reach the convective zone or the surface.
TEMPERATURE GRADIENT
The temperature in the radiative zone decreases with increasing distance from the core. This temperature gradient is essential for driving the flow of energy. Because of this gradient, photons gradually lose energy as they travel outward. The cooler outer layers of the Sun emit lower-energy, visible and infrared photons that eventually reach the surface and are radiated into space as sunlight.
RADIATIVE EQUILIBRIUM
The radiative zone is in a state of radiative equilibrium, where the energy absorbed in the lower layers is balanced by the energy radiated in the upper layers. This equilibrium maintains the Sun‘s stable temperature and prevents it from overheating or cooling too rapidly.
CONVECTIVE TRANSITION
The shift from the radiative zone to the convective zone in the Sun’s structure involves a change in the method of energy transfer. Here, instead of radiation, energy moves through the physical movement of hot plasma cells, known as convection cells. This transition takes place at the outer boundary of the radiative zone, where the temperature gradient steepens sufficiently for convection to take precedence as the primary mode of energy transfer within the Sun’s structure.
CONVECTIVE ZONE
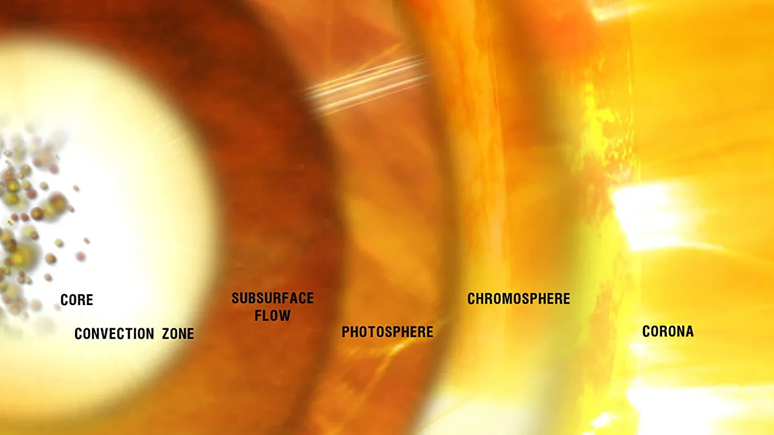
In the hierarchy of the Sun’s structure, the convective zone holds significant importance. Positioned above the radiative zone and beneath the Sun‘s visible surface, the photosphere, it serves as a vital intermediary. In contrast to the radiative zone’s reliance on radiation, the convective zone hinges on the dynamic movement of heated plasma to effectively convey heat and energy.
POSITION AND EXTENT
In the framework of the Sun’s structure, the convective zone is positioned directly above the radiative zone and spans from about 70% of the Sun’s radius to the region just below the photosphere, which constitutes the Sun’s visible surface.
ENERGY TRANSPORT MECHANISM
In a convective cell, hot plasma rises due to its lower density, carrying energy from the deeper layers toward the surface. As the hot plasma reaches the top of a cell, it cools and loses some of its energy, causing it to become denser and sink back down. This sinking plasma warms up as it descends and eventually re-enters the base of the convective zone to begin another cycle.
MIXING OF ELEMENTS
Convection in the Sun‘s convective zone plays a crucial role in mixing elements. It brings elements synthesized in the core to the surface, where they can be observed in the spectrum of sunlight. This mixing process enriches the surface layers with heavier elements such as carbon, nitrogen, and oxygen.
GRANULATION
The surface appearance of the convective zone is characterized by a pattern of bright granules and darker intergranular lanes. This granular pattern is a visual manifestation of the underlying convective cells.
TEMPERATURE GRADIENT
The temperature in the convective zone decreases with increasing distance from the photosphere. This temperature gradient provides the necessary conditions for convection to occur.
ENERGY EXCHANGE WITH PHOTOSPHERE
In the hierarchy of the Sun’s structure, the convective zone interfaces directly with the photosphere, where energy is emitted into space as visible light and heat. Within this zone, as plasma ascends, it conveys energy towards the photosphere, playing a crucial role in the Sun’s structure.
PHOTOSPHERE

As a pivotal component of the Sun’s structure, the photosphere stands as its observable surface, releasing both visible light and heat into the expanse of space. Below are several noteworthy attributes and characteristics of this crucial layer of the Sun’s structure:
POSITION AND EXTENT
The photosphere is the outermost layer of the Sun’s structure. It extends from the Sun’s interior, specifically the convective zone, to the outermost layer known as the chromosphere.
VISIBLE LIGHT EMISSIONS
The photosphere emits visible light, making it the layer of the Sun that we can observe with our eyes (using proper solar viewing techniques and protection). It is the part of the Sun that appears as a bright, continuous disc in the daytime sky.
TEMPERATURE AND COLOUR
The photosphere has an average temperature of approximately 5,500 degrees Celsius (9,932 degrees Fahrenheit). This temperature corresponds to a yellow-white colour, which is why the Sun appears white when seen from space or with solar filters.
GRANULATION
The photosphere exhibits a granular pattern known as granulation. These granules are typically about 1,000 kilometers (621 miles) in diameter and are caused by the motion of hot plasma rising from the Sun’s interior (convection). Hot plasma rises in the center of a granule, while cooler plasma sinks along the edges.
SUNSPOTS
Sunspots are dark, cooler regions on the photosphere’s surface. They are caused by intense magnetic fields that inhibit the flow of heat from the Sun’s interior to the surface. Sunspots often occur in pairs with opposite magnetic polarity and can vary in size from small spots to larger, more complex groups. The number of sunspots on the Sun’s surface follows an approximately 11-year cycle, known as the solar cycle.
FACULAE
Faculae are bright, granular regions found near sunspots. They are associated with areas of increased magnetic activity and are slightly hotter than the surrounding photosphere. Faculae contribute to the Sun‘s overall brightness and are more apparent when the Sun is observed at longer wavelengths, such as in the ultraviolet.
RADIATION INTO SPACE
The photosphere radiates visible light and heat into space, which is essential for sustaining life on Earth through the process of photosynthesis and maintaining our planet’s climate.
VISIBLE FEATURES
The photosphere occasionally exhibits other features such as solar flares and prominences, which are the result of complex magnetic interactions between the photosphere and the layers above it, including the chromosphere and corona.
CHROMOSPHERE
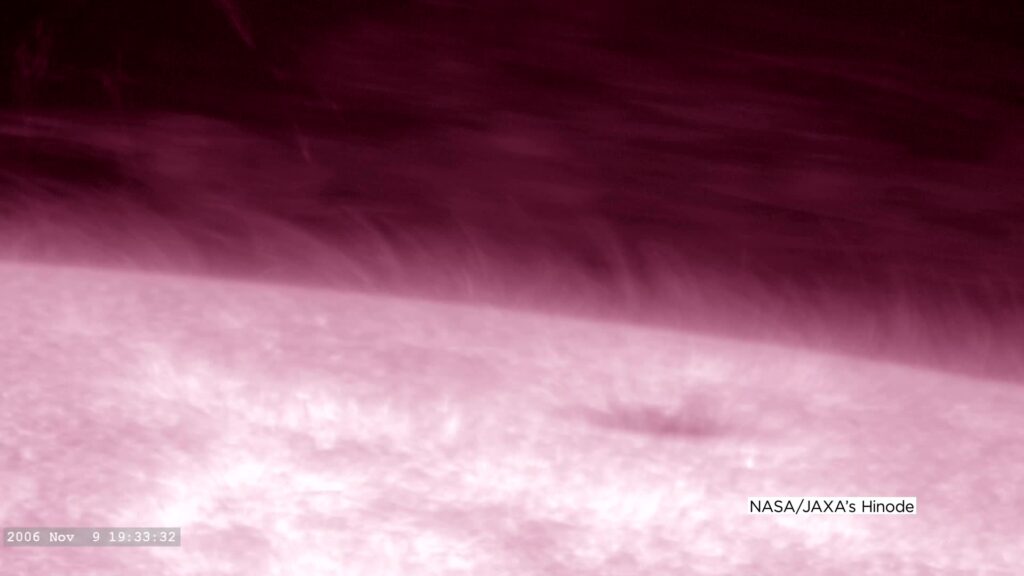
Within the Sun’s structure, the chromosphere holds a pivotal position in the solar atmosphere. Situated just above the photosphere and beneath the outermost layer, the corona, it derives its name from the reddish hue observed during solar eclipses. This distinctive coloration becomes visible when the photosphere is momentarily obscured, unveiling the outer layers of the chromosphere.
POSITION
The chromosphere lies immediately above the photosphere and extends to a height of several thousand kilometers above the Sun‘s surface. It is a relatively thin layer compared to the photosphere and the corona.
TEMPERATURE
The temperature in the chromosphere is relatively higher than the photosphere, though it is still cooler than the corona. It typically ranges from about 4,000 to 25,000 degrees Celsius (7,232 to 45,432 degrees Fahrenheit).
VISIBLE DURING ECLIPSE
The chromosphere is usually not visible because it is much fainter than the photosphere. However, during a total solar eclipse, when the Moon covers the bright photosphere, the chromosphere becomes briefly visible as a reddish ring surrounding the dark Moon.
FEATURES
The chromosphere exhibits a range of features and phenomena, including:
SPICULES
Spicules are short-lived, jet-like structures that protrude from the chromosphere into the corona. They are composed of hot, rising plasma and are often seen at the Sun’s limb (edge) during solar observations.
PROMINENCES
Prominences are massive, looping structures of relatively cool, dense gas suspended in the chromosphere. They can extend for thousands of kilometers and are often seen as bright, arching features during a total solar eclipse.
FILAMENTS
Filaments are similar to prominences but are seen against the bright disk of the Sun rather than the dark background of space. They appear as dark lines or ribbons and are caused by concentrations of cool, dense gas.
SOLAR FLARES
Some solar flares, explosive releases of energy and particles from the Sun, originate in the chromosphere. These flares result from the intense magnetic activity in this layer and can have significant impacts on space weather.
TRANSITION TO CORONA
Ascending beyond the chromosphere, we encounter the Sun’s structure’s outermost layer, the corona. This region, extending millions of kilometers into space, exhibits considerably higher temperatures. The process responsible for this temperature surge, termed the “coronal heating problem,” remains a vibrant area of study within the context of the Sun’s structure.
CORONA
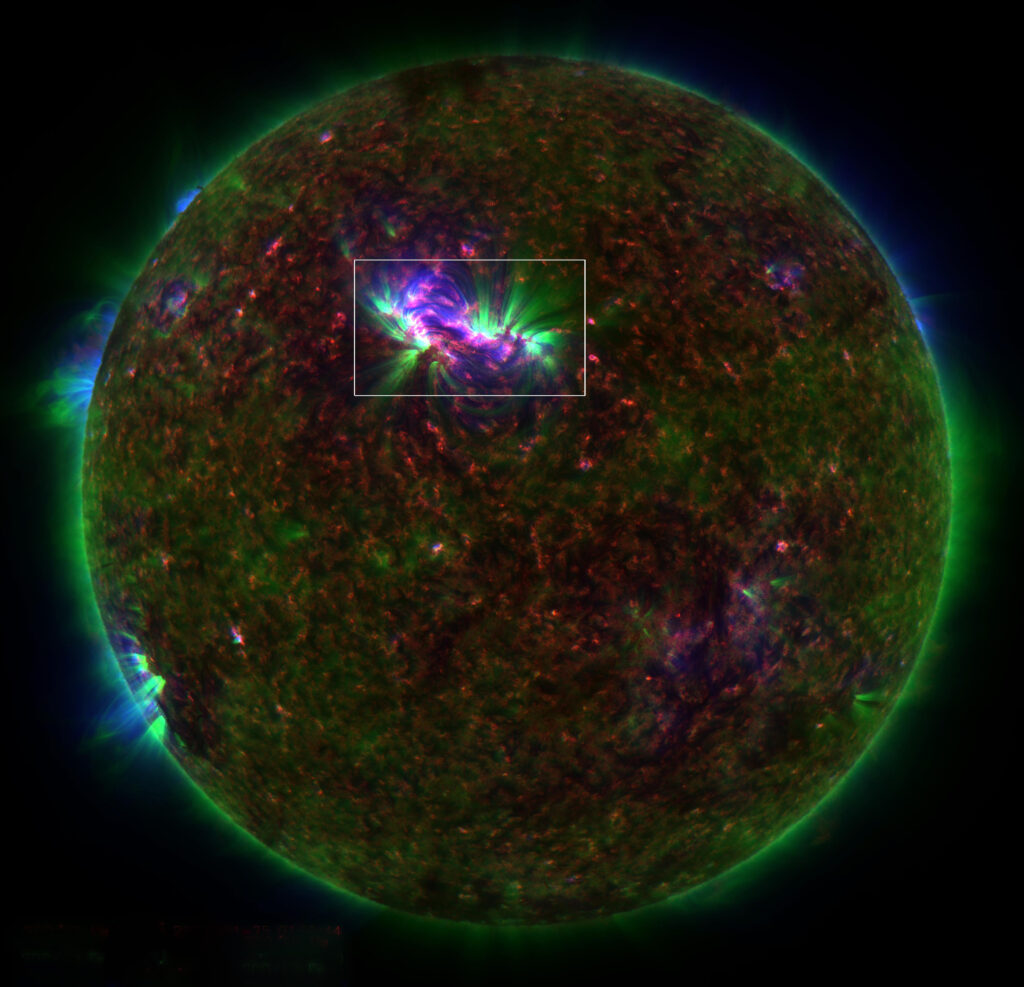
As an integral part of the Sun’s structure, the solar corona marks the outermost expanse of the Sun’s atmosphere, stretching millions of kilometers into the cosmic void. Within this region lies an incredibly hot and rarified plasma, boasting temperatures far surpassing those of the underlying layers, including the photosphere and chromosphere.
TEMPERATURE
The solar corona is paradoxically much hotter than the layers beneath it. While the photosphere has a temperature of around 5,500 degrees Celsius (9,932 degrees Fahrenheit), the temperature of the corona can reach millions of degrees Celsius (or Fahrenheit). This significant temperature difference between the corona and the photosphere is known as the “coronal heating problem,” and its precise cause is still a subject of research.
APPEARANCE
The corona is visible during total solar eclipses as a pearly-white halo surrounding the darkened disk of the Moon. It emits a faint, diffuse light that is typically overwhelmed by the brightness of the Sun‘s photosphere, making it challenging to observe with the naked eye.
CORONAL FEATURES
The corona exhibits various features and phenomena:
STREAMERS
These are elongated, bright structures that extend outward from the Sun’s surface. They follow the Sun’s magnetic field lines and can be seen during solar eclipses.
CORONAL LOOPS
Loop-like structures of hot plasma that follow the Sun’s magnetic field lines, often connecting regions of different magnetic polarity on the Sun’s surface.
CORONAL MASS EJECTIONS
Explosive releases of energy and particles from the corona can have a significant impact on space weather and can disrupt satellite communications and power grids on Earth.
SOLAR WIND
The corona is the source of the solar wind, a continuous flow of charged particles (mainly electrons and protons) that streams outward from the Sun and permeates the entire solar system.
MAGNETIC ACTIVITY
The corona is strongly influenced by the Sun’s magnetic field. It is the region where magnetic fields play a dominant role in shaping and heating the plasma. The interaction between magnetic fields in the corona can lead to the formation of coronal loops and other complex structures.
SOLAR OBSERVATIONS
To study the corona, scientists often use specialized instruments like coronagraphs and spectrographs that can block out the Sun’s bright disk, allowing the fainter corona to be observed. Space-based observatories like the Solar and Heliospheric Observatory (SOHO) and the Solar Dynamics Observatory (SDO) provide continuous observations of the Sun’s corona.
IMPACT ON SPACE WEATHER
The corona is closely associated with space weather phenomena. Coronal mass ejections and solar flares originating in the corona can have significant effects on Earth’s magnetosphere, causing geomagnetic storms, auroras, and disruptions to communication and navigation systems.
In conclusion, the Sun’s structure is a mesmerizing journey through the heart of our solar system’s central star. From the seething cauldron of the core, where nuclear fusion births light and heat, to the visible photosphere and the ethereal realms of the chromosphere and corona, the Sun’s structure is a testament to the beauty and complexity of the cosmos. These layers, each with its distinct characteristics and mysteries, offer a window into the Sun‘s inner workings and its influence on the space weather that shapes our solar system.
Understanding the Sun’s structure is not only a scientific endeavour but also a profound exploration of our connection to the cosmic dance of our nearest celestial neighbour. It reminds us of the vastness and wonder of the universe and our place within it, where the Sun reigns as a radiant beacon of light and life.