Stellar Titans
ยฉ NASA/ESA/STScI
Table of Contents
A giant star is characterized by its expansive radius relative to its mass and temperature, resulting in heightened brightness due to its correspondingly large radiating area. Positioned above the main sequence, designated as luminosity class V in the Yerkes spectral classification, on the HertzsprungโRussell diagram, giant stars correspond to luminosity classes II and III. The terms “giant” and “dwarf” were introduced by Ejnar Hertzsprung circa 1905 to describe stars of markedly different luminosity despite similar temperatures or spectral types.
Giant stars boast radii extending up to several hundred times that of the Sun, accompanied by luminosities ranging from 10 to a few thousand times that of the Sun. Stars even more luminous than giants are categorized as supergiants and hypergiants.
While a hot, luminous main-sequence star may sometimes be colloquially referred to as a giant, technically, any main-sequence star is classified as a dwarf star, irrespective of its size or luminosity.
FORMATION
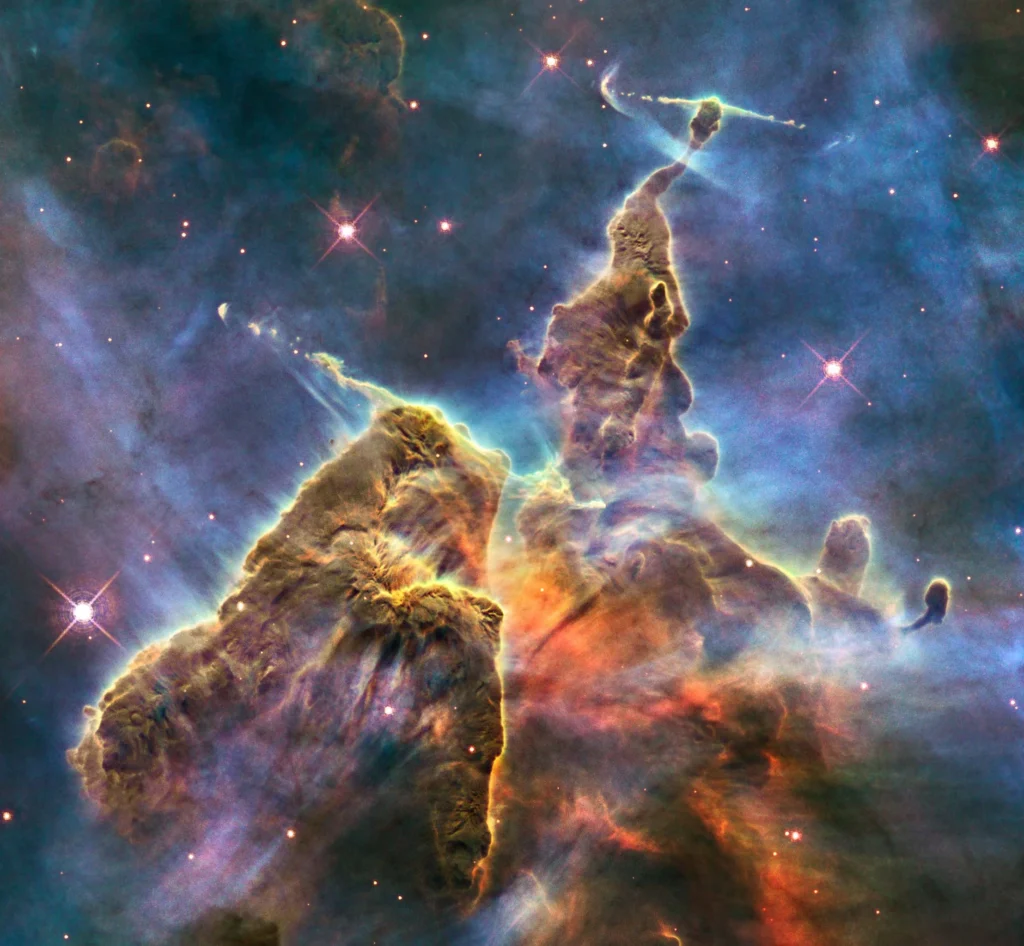
A giant star is formed when a star, like our Sun or larger, runs out of its hydrogen fuel. Inside a star, hydrogen atoms are combined together to form helium atoms, which create the energy that the star needs to resist the force of gravity that is trying to crush the star together and cause the star to light up.
Once all of the hydrogen has been turned into helium (after about 5 to 10 billion years, depending on the size of the star), there is no longer energy keeping the star from collapsing, so gravity takes over and pulls the star into itself. But the star only collapses so far that the helium atoms can begin to combine into carbon atoms. This process now provides enough energy to stop the star from collapsing and pushes the outermost layers of the star out, making the star much larger than it originally started. The star is now a Red Giant.
The formation of a giant star is a complex process that involves several stages. After all the hydrogen available for fusion at the core of a main sequence, the star has been depleted, the core begins to contract, and hydrogen now fuses to helium in a shell around the helium-rich core. The portion of the star outside the shell expands and cools, leading to the subgiant branch on the Hertzsprung-Russell diagram. The surface temperature of the star decreases, and the luminosity remains approximately constant. Eventually, the star will start to ascend the red giant branch on the Hertzsprung-Russell diagram, where the surface temperature remains approximately constant as the luminosity and radius increase drastically.
For a star with a mass of less than approximately 0.4 solar masses, it will not become a giant star because such stars have their interior thoroughly mixed by convection and continue fusing hydrogen until it is exhausted, at which point they become white dwarfs, composed chiefly of helium. This exhaustion, however, is predicted to take significantly longer than the lifetime of the universe up to now.
For a star with a mass greater than this lower limit, when it consumes all of the hydrogen in its core available for fusion, the core will begin to contract. Hydrogen now fuses to helium in a shell around the helium-rich core, and the portion of the star outside the shell expands and cools. The star’s position on the Hertzsprung-Russell diagram moves from the main sequence to the subgiant branch, and eventually, the star will ascend the red giant branch.
If the star’s mass, when on the main sequence, was below approximately 0.5 solar masses, it is thought that it will never attain the central temperatures necessary to fuse helium. It will therefore remain a hydrogen-fusing red giant until it eventually becomes a helium white dwarf.
If the star’s mass, when on the main sequence, was above approximately 0.5 solar masses, when the core temperature reaches approximately 10 8 K, helium will begin to fuse to carbon and oxygen in the core by the triple-alpha process. The energy generated by helium fusion causes the core to expand, reducing the pressure in the surrounding hydrogen-burning shell, which reduces its energy-generation rate. The star’s luminosity decreases, its outer envelope contracts again, and the star leaves the red giant branch. Its subsequent evolution will depend on its mass.
In summary, the formation of a giant star involves the depletion of hydrogen in the core of a main sequence star, leading to the contraction of the core and the expansion of the outer layers of the star. The star then ascends the red giant branch on the Hertzsprung-Russell diagram, where it remains for a significant portion of its life. The exact details of this process depend on the mass of the star, with lower-mass stars becoming red giants and higher-mass stars becoming supergiants or hypergiants.
TYPES OF GIANT STAR
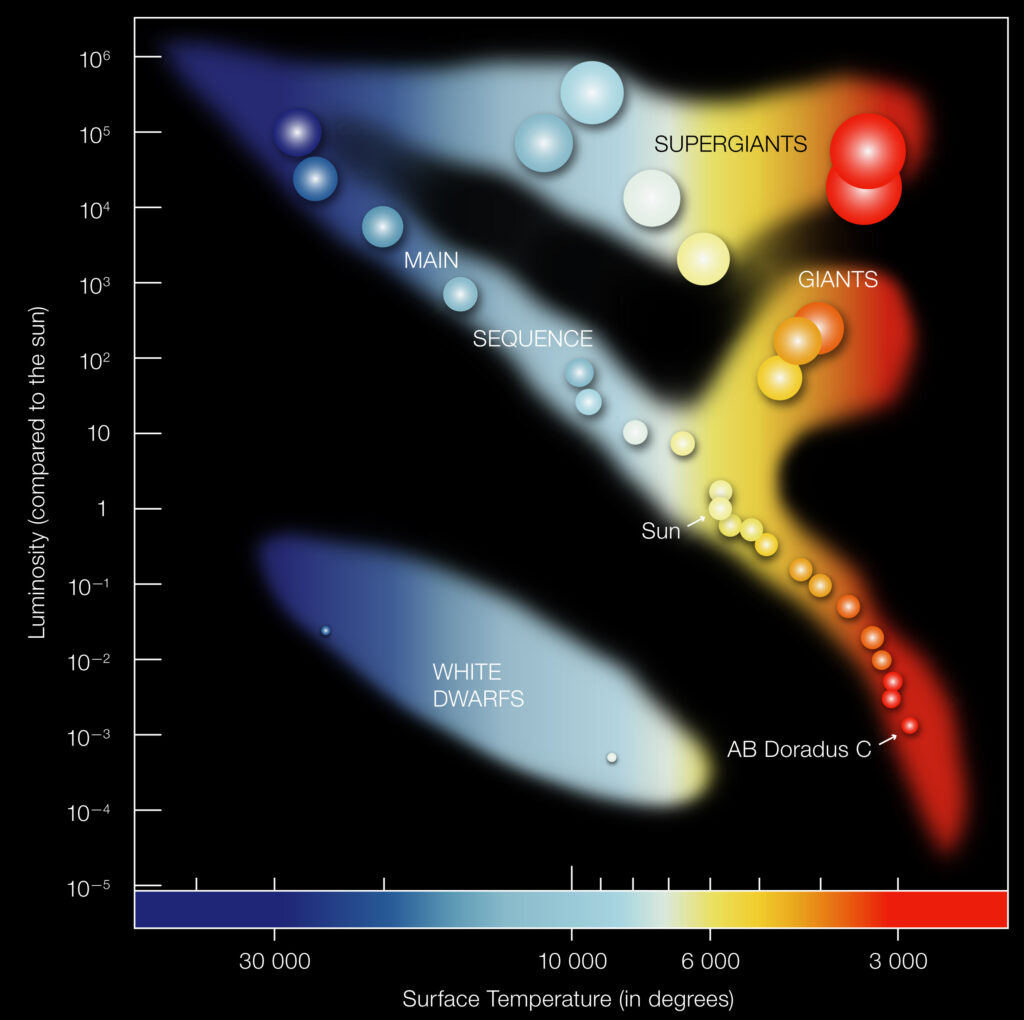
Giant stars come in several distinct types, each characterized by unique features and evolutionary pathways.
SUBGIANTS
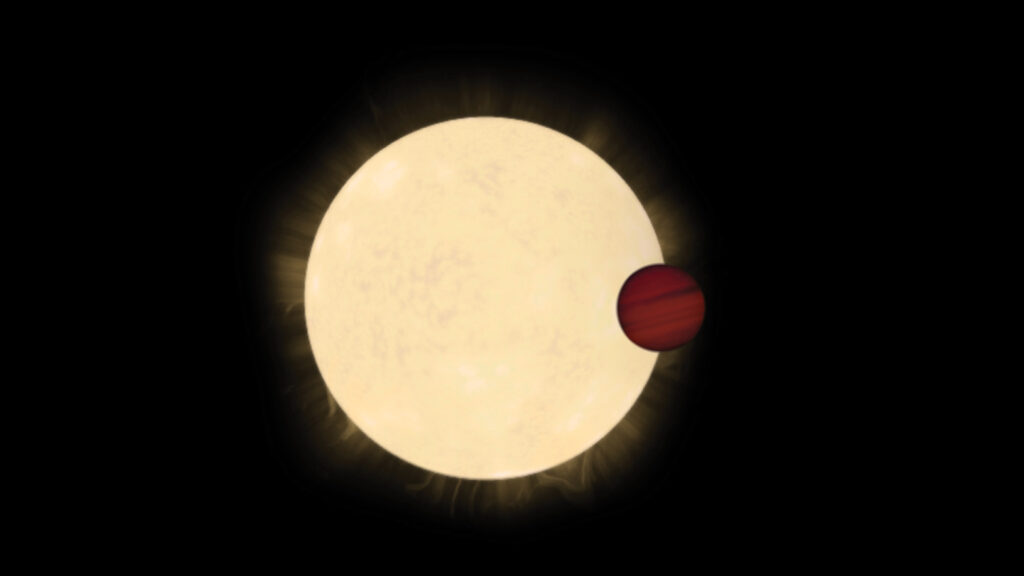
Subgiants, in the realm of stellar evolution, denote stars that have consumed the hydrogen at their cores, progressing from main-sequence status towards becoming giant stars. They possess greater luminosity than main-sequence stars of equivalent spectral classification, yet fall short of the brilliance exhibited by full-fledged giants. The term “subgiant” encompasses both a specific spectral luminosity classification and a pivotal stage in a star’s evolutionary journey. Positioned between main-sequence stars (classified as luminosity class V) and red giants (luminosity class III) in the Yerkes spectral classification system, subgiants occupy a distinctive niche.
Their characterization is notably evident in the Hertzsprung-Russell (H-R) diagram, where they trace a discernible trajectory. This diagram illustrates a prominent diagonal band representing the majority of stars, with red giants and subgiants forming significant clusters. Subgiants, distinguished by their reddish hue and expanded size relative to main-sequence counterparts of equivalent luminosity, are often detected in close binary systems, which provide conducive conditions for their identification.
The significance of subgiants extends to their role in elucidating stellar evolution, serving as intermediaries between main-sequence stars and red giants. Moreover, they serve as crucial reference points for asteroseismic investigations, facilitating the determination of fundamental properties such as mass, radius, and age.
BRIGHT GIANTS
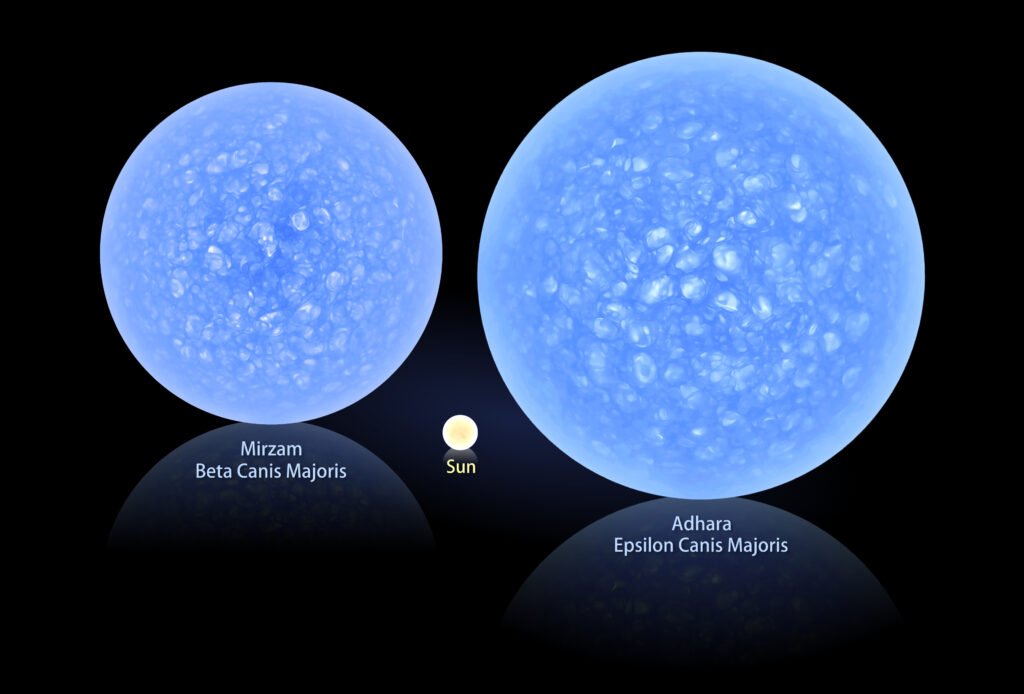
Bright giants, classified under luminosity class II, occupy an intermediate position between giant stars and supergiants concerning luminosity and spectral characteristics. Encompassing the entire spectral range from blue to red, they are distinguished by their placement on the Hertzsprung-Russell (H-R) diagram. Bright giants surpass both main-sequence stars and giant stars of comparable spectral type in brightness and size, and they outshine subgiants as well.
In the context of stellar evolution, bright giants play a significant role, marking a stage wherein stars have depleted hydrogen in their cores and commenced helium burning. They serve as pivotal reference points for discerning stellar properties such as mass, radius, and temperature.
Prominent examples of bright giant stars include Adhara, a blue luminary in Canis Major, the radiant yellow Sargas in Scorpius, and the scorching O-type star Mintaka, a constituent of Orion’s Belt. Additionally, there exist subcategories within the realm of bright giants, such as M-type bright giants, characterized by their spectral type M.
SUPER GIANTS
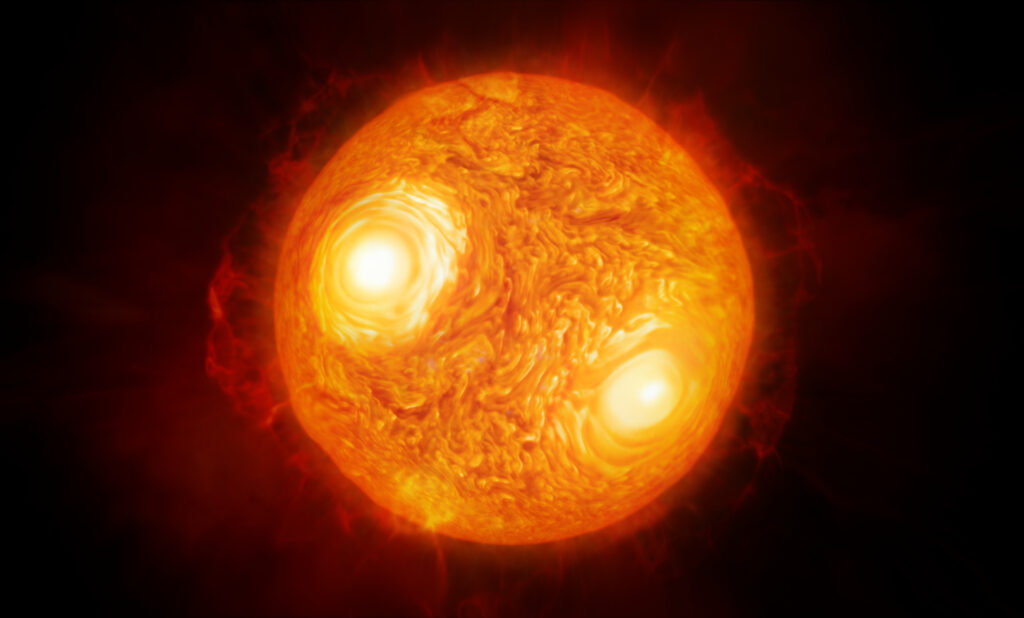
Supergiants stand out as some of the most massive and brilliantly luminous celestial bodies, occupying the upper echelons of the Hertzsprung-Russell diagram with absolute visual magnitudes ranging from approximately -6 to -12. Distinguishing features include their spectra, which exhibit distinctive lines indicative of high luminosity and low surface gravity, spanning the entire spectral range from youthful blue class O supergiants to mature red class M supergiants. Unlike their lower-mass counterparts, red supergiants boast sufficient mass to initiate fusion reactions of elements heavier than helium within their cores, persisting in this process until gravitational collapse occurs, often culminating in the formation of neutron stars or black hole remnants, typically following a core-collapse supernova event.
The most colossal stars, exceeding approximately 100 solar masses, undergo minimal displacement from their initial position as O-type main-sequence stars, progressing through various stages such as supergiants, WNh-, WN-, and potentially WC- or WO-type stars before climaxing in supernova eruptions. The presence of supergiants maintaining hydrogen fusion in their cores necessitates a nuanced definition, as they represent massive entities with augmented size and luminosity due to the accumulation of fusion byproducts, albeit with residual hydrogen content. Supergiants are integral to comprehending stellar evolution and wield substantial influence in the genesis of elements within the cosmos.
HYPER GIANTS
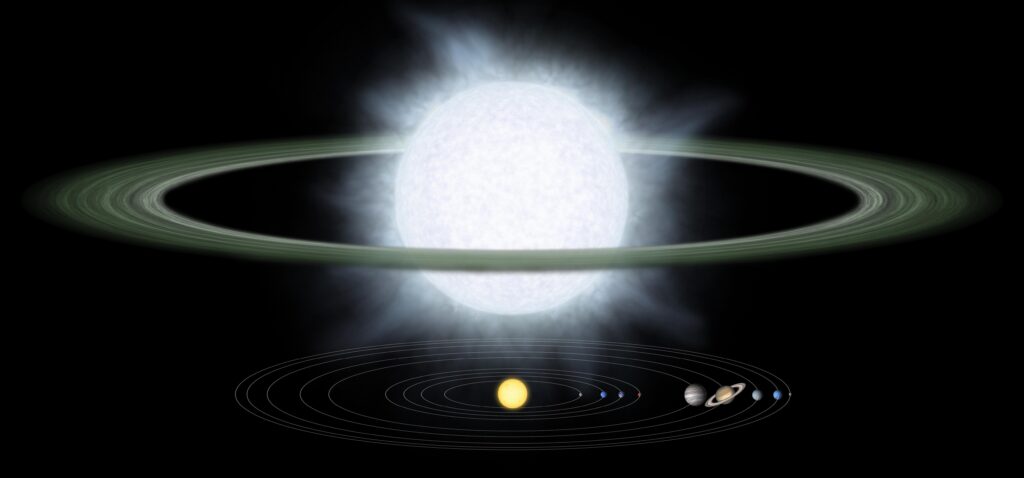
Hypergiants stand as the pinnacle of stellar massiveness and luminosity, boasting absolute visual magnitudes ranging from approximately -8 to -12, surpassing even the brilliance of supergiants. Their spectral signatures reveal pronounced lines of hydrogen, helium, and heavier elements, reflective of their towering luminosity and diminished surface gravity. These celestial behemoths are exceedingly rare, with only a scant few identified within the confines of the Milky Way, notable examples including Eta Carinae and P Cygni.
Crucial to unravelling the intricacies of massive stellar evolution, hypergiants epitomize a fleeting yet pivotal stage in the existence of the most massive stars in the cosmos. Moreover, they play a vital role in elucidating the chemical evolution of galaxies, capable as they are of generating and disseminating copious quantities of heavy elements into the interstellar medium.
Hypergiants are also renowned for their extreme variability, with fluctuations in brightness spanning several magnitudes over mere years. This variability is attributed to monumental surface eruptions that can expel vast quantities of material into space. Such eruptions may engender the formation of intricate nebulae surrounding the star, offering insights into its historical trajectory and evolutionary narrative.
RED GIANTS
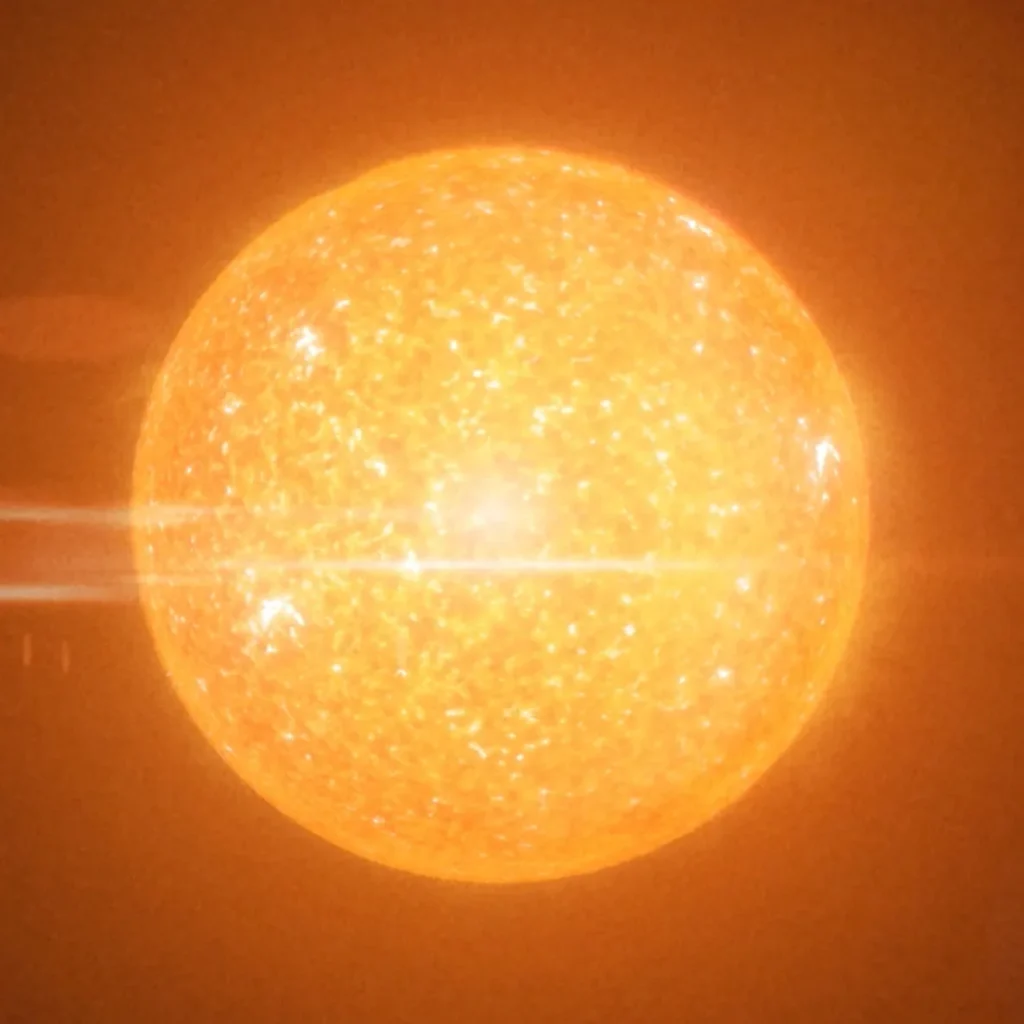
Red giants, the luminous titans of stellar evolution, emerge as stars of modest to intermediate-mass traversing the twilight phase of their cosmic journey. With expansive radii spanning tens to hundreds of times that of our Sun, and surface temperatures hovering around 5,000 K (4,700 ยฐC; 8,500 ยฐF) or lower, they exude a warm yellowish-orange hue, occasionally tinted with hues of reddish-orange, typified by spectral types K and M, and occasionally G, along with class S stars and the majority of carbon stars.
Energy production within red giants varies contingent upon their evolutionary stage. Predominantly, those situated on the red-giant branch (RGB) sustain hydrogen-to-helium fusion within a shell. Conversely, red-clump stars inhabiting the cooler realms of the horizontal branch facilitate the transformation of helium into carbon at their cores through the triple-alpha process. Meanwhile, asymptotic-giant-branch (AGB) stars harbor a helium-burning shell enveloping a degenerate carbon-oxygen core, alongside a hydrogen-burning shell further afield.
Integral to unravelling the tapestry of stellar evolution, red giants epitomize a twilight epoch for stars of modest to intermediate mass. Moreover, their significance extends to galactic chemical evolution, as they serve as catalysts for the production and dissemination of substantial quantities of heavy elements into the interstellar milieu.
In a cosmic symphony of transitions, our own Sun is destined to join the ranks of red giants in approximately 5 billion years. During this metamorphosis, it will burgeon to over 200 times its present diameter, ensnaring Mercury, Venus, and conceivably Earth within its expanded embrace. Shedding 38% of its mass in the process, it will ultimately condense into a white dwarf, marking the culmination of its stellar odyssey.
YELLOW GIANTS
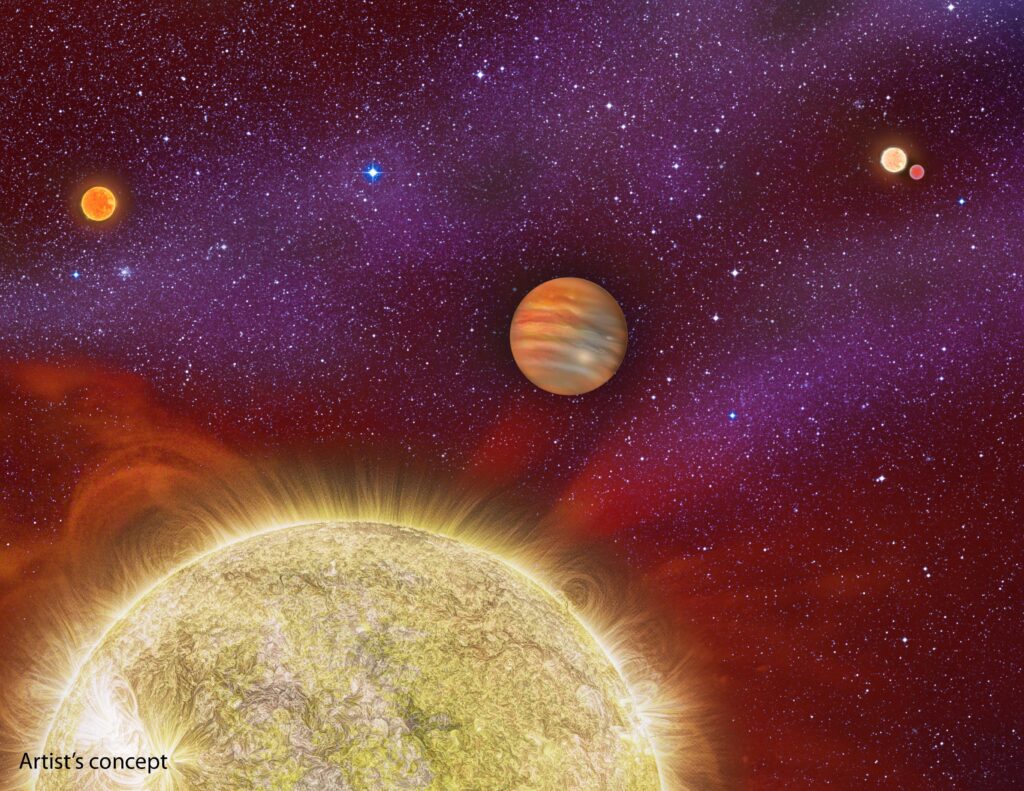
Yellow giants, stellar entities bearing F or G spectral designations, manifest with masses spanning from 0.5 to 11 times that of the Sun. They may also manifest in a supergiant guise, distinguished by luminosity classifications of Ia or Ib. Relatively dwarfed by their red supergiant counterparts, yellow giants are discerned via their spectra, characterized by prominent hydrogen lines that diminish across F and G classes and are nonexistent within K-class stars.
Despite varying masses, these stars typically exhibit temperatures ranging from 4,000K to 7,000K and have transitioned from their main-sequence status after depleting hydrogen within their cores. Notably, some yellow giants undergo a transient transition from red giants, termed a “blue loop,” and may exhibit pulsations akin to regular Cepheid variables. While the likelihood of yellow supergiants precipitating supernovae is minimal, certain post-red supergiant yellow supergiants may undergo collapse, instigating core-collapse supernovae.
ORANGE GIANTS
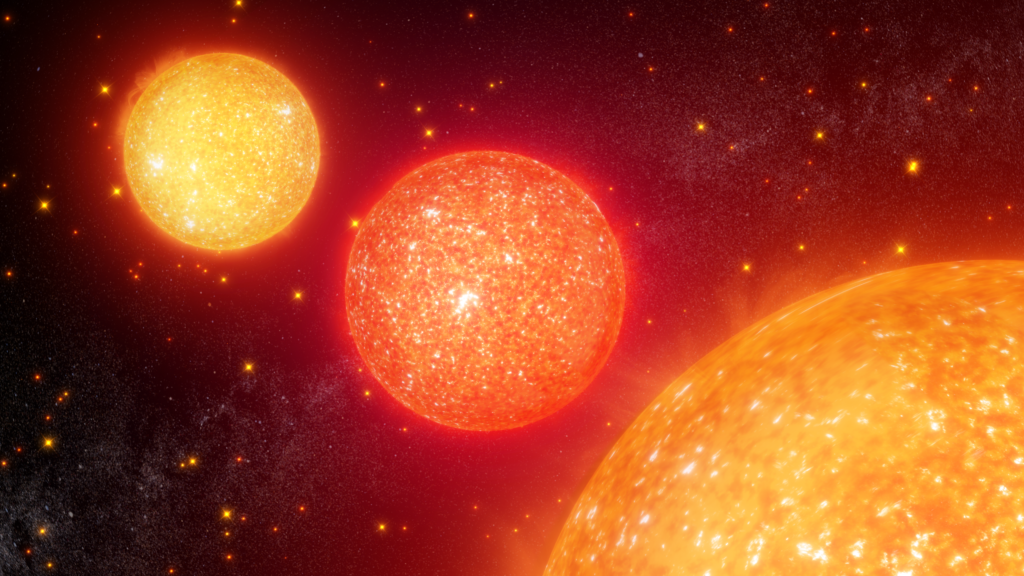
Orange giants, distinguished by their size and luminosity surpassing that of the Sun yet falling short of the grandeur of red giants, epitomize a late evolutionary phase. Their distinctive orange hue stems from a surface temperature hovering around 4,000 to 5,000 degrees Celsius. Having depleted the hydrogen within their cores, they initiate helium fusion, prompting the outer layers to expand and cool, thereby enlarging the star and reducing its temperature. Relatively scarce, orange giants mark a fleeting juncture in a star’s trajectory before transitioning into red giants or white dwarfs. Vital to comprehending stellar evolution, they signify an intermediary stage bridging main-sequence stars and red giants.
BLUE GIANTS

Blue giants, denoted by their spectral classification of O or B and a luminosity class of III, represent a category of massive stars. Their luminosity, approximately 10,000 times that of the Sun, accompanies a scorching surface temperature exceeding 20,000 kelvin (K). Predominantly congregated within OB associations, these celestial luminaries, despite their moniker, typically do not herald the terminal phases of their existence but rather signify a transitional epoch preceding their metamorphosis into either bright giants or supergiants.
Distinct from blue supergiants or conventional hydrogen-fusing O to B stars, blue giants stand as exceptionally hot, luminous, and expansive entities, boasting a mass range spanning 5 to 10 times that of the Sun. Ongoing research endeavours continually illuminate our understanding of these enigmatic stars, which, despite their rarity, offer profound insights into the cosmic tapestry, with only a scant few examples identified within the Milky Way.