Explosive Finale of Stellar Evolution
ยฉย NASA Goddard
Table of Contents
A supernova marks the explosive finale of a star’s life or when a white dwarf ignites into runaway nuclear fusion, erupting in a dazzling burst of light. The original star, known as the progenitor, either collapses into a neutron star or black hole or disintegrates, forming a diffuse nebula. These cosmic eruptions can briefly outshine entire galaxies and emit more energy than our Sun will emit throughout its lifetime.
The term “supernova” originates from “nova” (Latin for “new”), referring to another type of exploding star. Supernovae share characteristics with novae: both undergo a rapid and intense brightening lasting weeks, followed by a gradual dimming. Spectroscopic analysis reveals blue-shifted emission lines, indicating the expulsion of hot gases. However, a supernova explosion, unlike a nova outburst, is a cataclysmic event for a star, effectively terminating its active energy-generating phase. When a star “goes supernova,” vast amounts of matter, equivalent to several solar masses, may be propelled into space with such energy that the exploding star outshines its entire home galaxy.
Supernova explosions unleash vast quantities of radio waves, X-rays, and cosmic rays, with some gamma-ray bursts linked to these cataclysmic events. Furthermore, supernovae are significant contributors to the production of heavy elements essential for the formation of celestial bodies like Earth. Spectral analyses reveal elevated levels of these heavier elements, suggesting their synthesis during the explosive phase. The expanding shell of a supernova remnant persists until reaching an advanced stage, gradually dispersing into the surrounding interstellar medium.
TYPES OF SUPERNOVA
Supernovae are categorized by astronomers based on their light curves and the presence of specific absorption lines in their spectra. If a supernova spectrum reveals lines of hydrogen, it falls under the Type II classification; otherwise, it belongs to Type I. Each of these classifications further branches into subdivisions, which depend on additional elements’ line presence in the spectrum or the light curve’s shape.
TYPE I SUPERNOVA
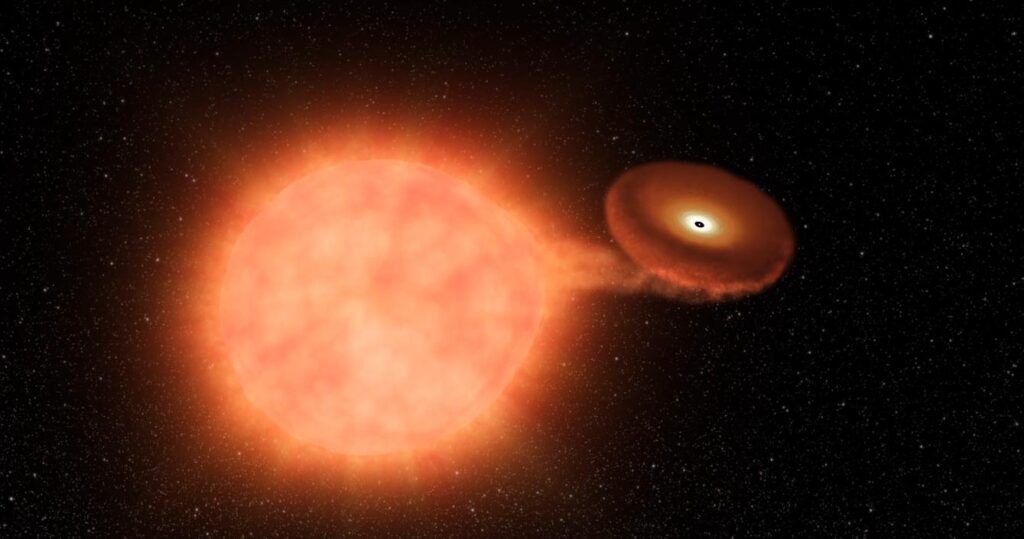
Type I supernovae are a class of supernovae that are characterized by the lack of hydrogen in their spectra. This class is further divided into three subcategories:
TYPE Ia
Type Ia supernovae manifest in binary star systems where one star is a white dwarf. Over time, the white dwarf accumulates material from its companion until it reaches the Chandrasekhar mass limit of 1.44 solar masses. Upon reaching this critical point, the white dwarf’s core undergoes a runaway thermonuclear explosion, obliterating the star entirely. Renowned for their uniform peak brightness, Type Ia supernovae serve as invaluable “standard candles” for gauging cosmic distances. Remarkably, they are found in various galaxy types, dispelling the notion that they are exclusive to regions of active star formation.
TYPE Ib
Type Ib supernovae stem from the collapse of massive stars that have shed their outer hydrogen layers, leaving behind a helium-rich core. Spectroscopic analysis of Type Ib supernovae reveals prominent helium signatures but minimal to nonexistent hydrogen lines. These explosions are associated with progenitor stars of at least 25 solar masses, indicating their immense size and mass. Unlike Type Ia supernovae, which involve white dwarfs in binary systems, Type Ib supernovae arise from the demise of single massive stars, showcasing the diverse pathways leading to these cataclysmic cosmic events.
TYPE Ic
Type Ic supernovae, akin to Type Ib, originate from progenitor stars that have shed both their hydrogen and helium layers. In the spectra of Type Ic supernovae, the absence or minimal presence of hydrogen and helium lines distinguishes them from other supernova types. These explosions typically arise from massive stars that have undergone extensive mass loss processes, leaving behind stripped cores rich in heavier elements. The dearth of hydrogen and helium signatures underscores the unique evolutionary pathways of these progenitor stars. Such observations deepen our understanding of the diverse mechanisms leading to the cataclysmic demise of massive stars in the universe.
TYPE II SUPERNOVA
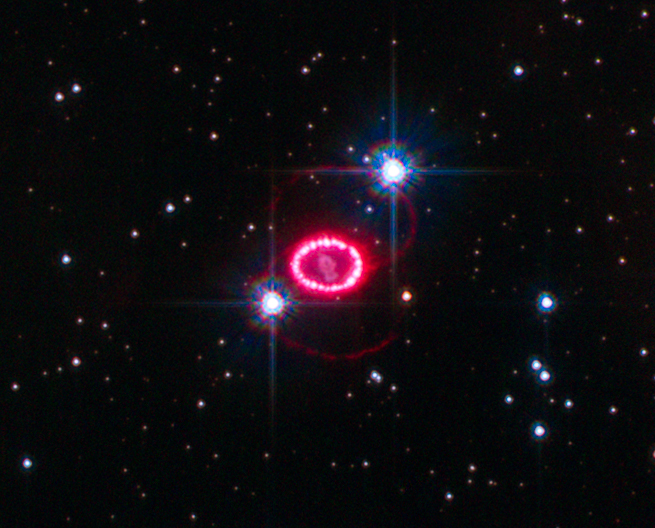
Type II supernovae constitute a category of stellar explosions distinguished by the prominent presence of hydrogen in their spectral signatures. These cosmic events stem from the rapid gravitational collapse followed by a powerful explosion of massive stars, usually possessing a minimum mass of 8 times that of the Sun.
ย TYPE II-P
Type II-P supernovae exhibit a distinctive feature in their light curvesโan initial “plateau” phase. This plateau arises from a sustained release of energy following the explosion, resulting in a relatively stable luminosity over a certain period. This characteristic plateau phase, observed in the light curve, provides valuable insights into the physical processes occurring within the supernova.
TYPE II-L
Type II-L supernovae differ from their II-P counterparts in their post-explosion behaviour. Instead of exhibiting a plateau phase, the luminosity of Type II-L supernovae experiences a linear decline over time. This distinctive decline in luminosity following the initial explosion provides astronomers with additional clues about the nature and dynamics of these cosmic events.
TYPE III,IV,V SUPERNOVA
Zwicky introduced new classifications for supernovae based on a handful of instances that defied the conventional parameters of type I or type II supernovae. For instance, SN 1961i in NGC 4303 became the archetype of the type III supernova category, distinguished by its broad light curve maximum and slow development of hydrogen Balmer lines in the spectrum.
Meanwhile, SN 1961f in NGC 3003 represented the sole example of the type IV class, exhibiting a light curve akin to a type II-P supernova but with weak hydrogen emission lines contrasting hydrogen absorption lines. SN 1961V in NGC 1058, dubbed as type V, stood out as an enigmatic faint supernova or impostor, characterized by a prolonged rise to brightness, an extended maximum phase lasting many months, and an unconventional emission spectrum reminiscent of the Eta Carinae Great Outburst. Additionally, supernovae observed in M101 (1909) and M83 (1923 and 1957) were suggested as potential type IV or type V candidates.
Today, these categories are generally regarded as peculiar type II supernovae (IIpec), encompassing a broader array of similar events. Despite numerous discoveries, debates persist regarding whether SN 1961V truly qualified as a supernova following a LBV (Luminous Blue Variable) outburst or if it was an impostor.
CORE COLLAPSE
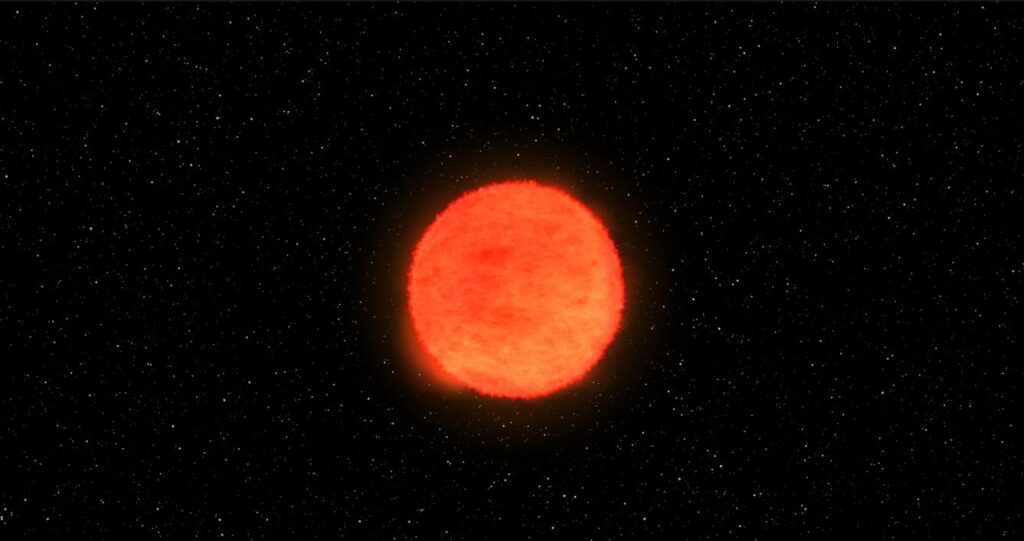
The core collapse of a massive star is the key event that triggers a supernova explosion. This process occurs in stars with masses greater than about 8 times the mass of the Sun.
The sequence of events is as follows:
- Fusion in the core: As a massive star ages, its core undergoes successive stages of nuclear fusion, burning progressively heavier elements up to iron.
- Core composition: The core of the star is composed primarily of iron and nickel, with concentric shells of progressively lighter elements surrounding it.
- Core instability: Once the core is dominated by iron, further fusion cannot occur, as iron has the highest binding energy of all elements. This causes the core to become unstable and begin to collapse under its own gravity.
- Rapid collapse: As the core collapses, it reaches extremely high densities and temperatures, up to 100 billion Kelvin. This causes the core to implode at velocities up to 23% of the speed of light.
- Neutron and neutrino production: The rapid compression causes the electrons in the core to combine with protons via inverse beta decay, producing neutrons and neutrinos. This releases an enormous amount of energy, around 10^46 joules, in just 10 seconds.
- Rebound and shock wave: The collapse is halted by the repulsive nuclear force and neutron degeneracy pressure. This causes the core to rebound outward, forming a shockwave that propagates through the star’s outer layers.
- Supernova explosion: The shockwave, combined with the extreme temperatures and pressures, is powerful enough to disrupt the star’s outer layers, ejecting them into space at escape velocity, resulting in the supernova explosion.
The final remnant of the core collapse can be either a neutron star or a black hole, depending on the initial mass of the star.
Cause of collapse | Progenitor star approximate initial mass (solar masses) | Supernova type | Remnant |
---|---|---|---|
Electron capture in a degenerate O+Ne+Mg core | 9-10 | Faint II-P | Neutron Star |
10-25 | Faint II-P | Neutron Star | |
25โ40 with low or solar metallicity | Normal II-P | Black hole after fallback of material onto an initial neutron star | |
Iron core collapse | 25โ40 with very high metallicity | II-L or II-b | Neutron star |
40โ90 with low metallicity | None | Black hole | |
โฅย 40 with near-solar metallicity | Faint Ib/c, or hypernova with gamma-ray burst (GRB) | Black hole after fallback of material onto an initial neutron star | |
โฅย 40 with very high metallicity | Ib/c | Neutron star | |
โฅย 90 with low metallicity | None, possible GRB | Black hole | |
Pair instability | 140โ250 with low metallicity | II-P, sometimes a hypernova, possible GRB | No remnant |
Photodisintegration | โฅย 250 with low metallicity | None (or luminous supernova?), possible GRB | Massive black hole |
TYPE II SUPERNOVA
Stars with initial masses below approximately 8 solar masses (Mโ) never develop cores large enough to undergo collapse, eventually shedding their atmospheres to form white dwarfs. On the other hand, stars with masses of at least 9 Mโ follow a complex evolutionary path, progressively fusing heavier elements at higher temperatures within their cores. This leads to a layered structure akin to an onion, with various elements burning in concentric shells. While often depicted as having an iron core, the least massive supernova progenitors possess oxygen-neon(-magnesium) cores. These super-asymptotic giant branch (super-AGB) stars may constitute the majority of core collapse supernovae, although they are less luminous and thus less frequently observed compared to their more massive counterparts.
During the supergiant phase, if the core collapse occurs while the star retains a hydrogen envelope, it results in a type II supernova. The rate of mass loss in luminous stars is influenced by factors like metallicity and luminosity. Highly luminous stars with near-solar metallicity typically lose their hydrogen before experiencing core collapse, thus skipping the type II supernova phase. Conversely, at low metallicity, all stars retain their hydrogen envelopes until core collapse, though sufficiently massive ones directly collapse into black holes without producing observable supernovae.
Stars with initial masses up to approximately 90 times that of the Sun, or slightly less at high metallicity, culminate in type II-P supernovae, the most commonly observed type. In regions of moderate to high metallicity, stars near the upper end of this mass range lose most of their hydrogen before core collapse, resulting in type II-L supernovae. At extremely low metallicity, stars within the range of 140โ250 Mโ undergo core collapse due to pair instability while still possessing a hydrogen atmosphere and an oxygen core, leading to supernovae exhibiting type II characteristics but with substantial ejection of nickel-56 and high luminosity.
TYPE Ib and Ic SUPERNOVA
Similar to type II supernovae, these supernovae originate from massive stars experiencing core collapse. However, unlike the progenitors of type II supernovae, the stars evolving into types Ib and Ic supernovae have shed most of their outer (hydrogen) envelopes due to intense stellar winds or interactions with a companion. These stars, known as WolfโRayet stars, occur in environments with moderate to high metallicity, where continuum-driven winds lead to significant mass-loss rates. Despite this, observations of type Ib/c supernovae don’t entirely align with the presence of WolfโRayet stars. Alternate theories suggest binary interactions stripping stars of their hydrogen, offering a more fitting explanation for these core collapse supernovae, albeit with no observed binary helium stars matching the criteria.
Type Ib supernovae, more prevalent, originate from WolfโRayet stars of type WC, retaining helium in their atmospheres. In a specific mass range, stars evolve further to become WO stars, with minimal remaining helium, serving as progenitors for type Ic supernovae.
A small percentage of type Ic supernovae are linked to gamma-ray bursts (GRBs). However, it’s theorized that any hydrogen-stripped type Ib or Ic supernova could generate a GRB, contingent on geometric circumstances. The mechanism involves jets from the rapidly spinning magnetar formed within the collapsing core, transferring energy to the expanding outer shell, resulting in a super-luminous supernova.
OBSERVATIONS
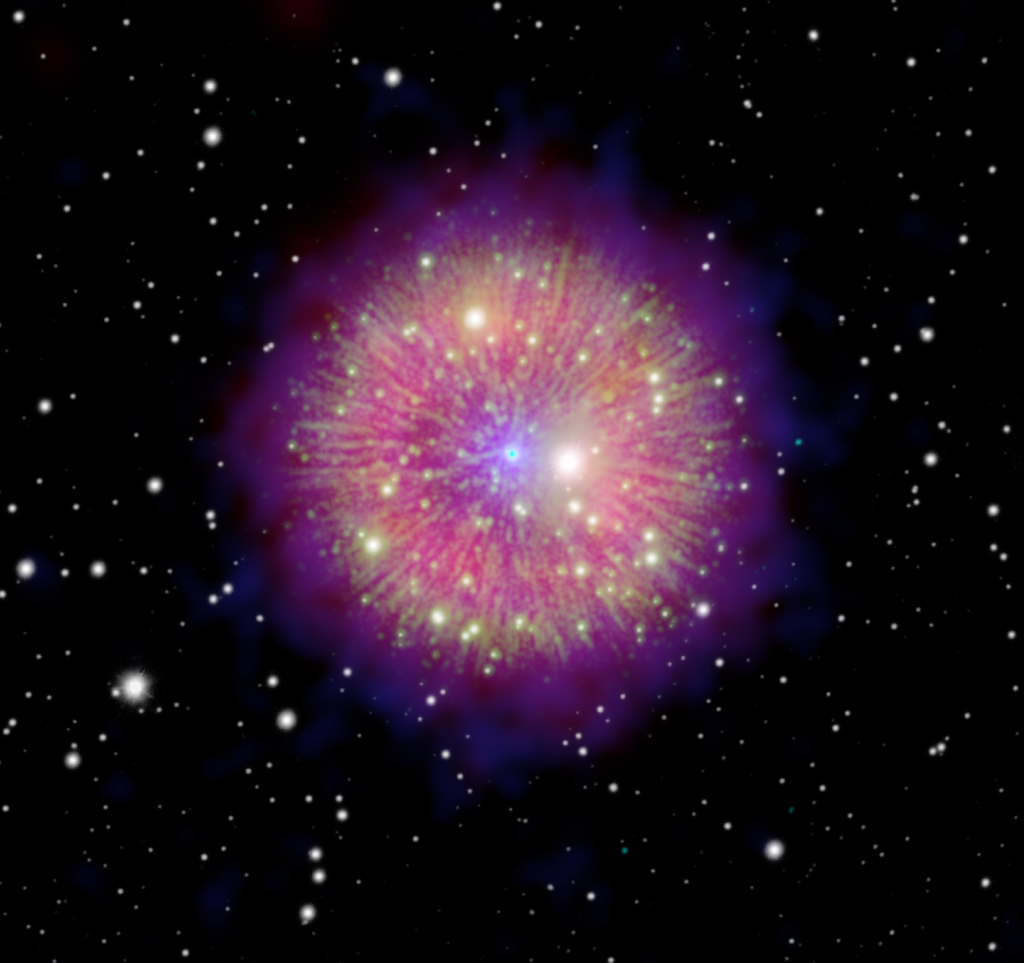
Recorded supernova observations trace back to 185 AD when Chinese astronomers documented a “new star” visible for 8 months. This event stands as the earliest confirmed supernova observation by humans. Modern astronomers have identified the remnant of this supernova, known as RCW 86.
Additionally, ancient Greek and Roman astronomers documented sudden appearances of bright stars in the sky. A significant instance occurred in 1054 AD when a supernova event created the Crab Nebula. This phenomenon was recorded by astronomers from various cultures, including Chinese, Japanese, Arab, and Native American astronomers.
EARLY MODERN ERA
- Tycho’s Supernova (SN 1572): In 1572, the Danish astronomer Tycho Brahe observed a new star in the constellation Cassiopeia. This event, now known as Tycho’s supernova, challenged the prevailing Aristotelian view of the unchanging heavens and provided evidence against the notion of celestial perfection.
- Kepler’s Supernova (SN 1604): Johannes Kepler observed another supernova in 1604, now known as Kepler’s Supernova. This event, along with Tycho’s supernova, provided crucial evidence against the Aristotelian cosmology and supported the emerging heliocentric model proposed by Copernicus.
MODERN OBSERVATIONS AND UNDERSTANDING
- Advances in Telescopes and Technology: In the 20th century, the field of supernova discovery expanded to other galaxies with the development of telescopes. Successful models of supernova behaviour have been developed, and the role of supernovae in the star formation process. They categorized supernovae based on their spectra and light curves, leading to the classification of Type I and Type II supernovae.
- Supernovae as Cosmic Yardsticks: In the 20th century, supernovae became invaluable tools for measuring cosmic distances. The discovery of Type Ia supernovae as standard candles led to the realization that the universe’s expansion is accelerating, a discovery awarded the Nobel Prize in Physics in 2011.
In recent times, several noteworthy supernovae have captured the attention of astronomers. Among them is SN 1987A, the first supernova observed in modern times. This event presented a unique opportunity to validate theories regarding supernova formation.
More recently, the discovery of the “zombie star” iPTF14hls has perplexed scientists by showcasing multiple supernova-like explosions occurring over several years. This phenomenon challenges our conventional understanding of stellar death and poses intriguing questions about the dynamics of such cosmic events.